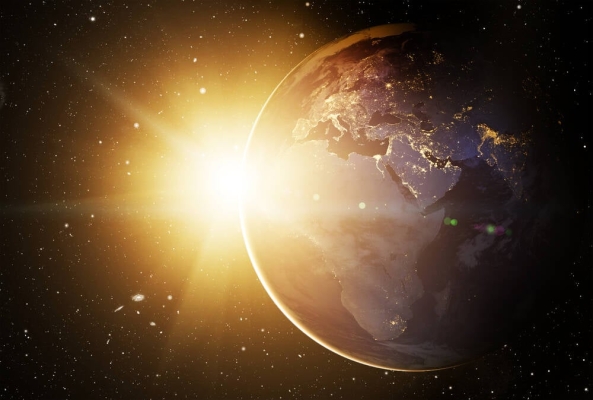
Scientists mull Solar Radiation Management – a potential climate-change stop-gap
In-depth At the American Geophysical Union annual meeting (AGU23) in San Francisco the other week, the 25,000-plus science folks in attendance pretty much all agreed on one unequivocal fact: the Earth is warming and it’s warming quickly. Discussions centered not on “if” — that’s been settled — but on how to best measure that rise, how to best model it, and what best to do about it.
The most straightforward answer to that latter question, of course, has also been settled: Stop pumping the troposphere — the lower level of the atmosphere in which we all live and breathe — full of greenhouse gasses (GHGs) such as carbon dioxide (CO2 — abundant and long-lasting, though relatively weak) and methane (CH4 — far less abundant and short-lasting, but quite strong).
One gigantic problem exists, however: The world’s economies currently largely run on GHG–producing fossil fuels. To unceremoniously and immediately yank those life-enhancing energy sources out from under eight billion people would cause untold disruption, suffering, and strife. Investigations are therefore underway about what if anything to do to slow down global warming long enough to allow the inevitable transition to renewable energy — wind, solar, nuclear, geothermal, hamsters in wheels, whatever — to be reasonably successful.
Enter geoengineering. Essentially, this catch-all set of techniques is about hacking Earth systems in such a way as to defeat — even if only temporarily — global warming and its evil twin climate change. After all, atmospheric (as opposed to orbital, precession, and other factors) global warming is caused by the simple physics of the Sun’s short-wave energy bouncing off the Earth’s surface as long-wave infrared radiation, then being absorbed and reradiated by loosely bound gas molecules such as CO2 and CH4, which then warm the troposphere and cool the stratospheric level above it, as has been incontrovertibly measured for decades.
As any climatologist will tell you, natural global warming is A Good Thing™. Simple physics (such as the Stephan-Boltzmann Law, for you aspiring climate geeks) proves the Earth would average about a frigid -15°C without our planet’s blanket of GHGs. Thanks to our GHG coverlet, we average around 15°C. The problem is that since we’re rapidly adding to those GHGs, the Earth’s global temperature is also rapidly rising. That’s a problem.
A favorite among geoengineering techniques, as a climate change mitigation, is Solar Radiation Management (SRM), which suggests that if the Sun is heating us up, why not just block its rays from beating down so furiously on our defenseless li’l planet? Wouldn’t, couldn’t, and perhaps shouldn’t a concerted effort be made to find a way to block the sun and buy us some time while we kick our fossil-fuel addiction?
Ah, if it were only that easy.
Channeling Mr Burns
A broad range of SRM solutions have been run up the proverbial flagpole. For example, sun-blocking mirrors in space have been proposed, including one “about the size of Brazil”, or perhaps a swarm of 800,000 metre-sized reflectors. Other ideas include making the ocean more reflective by injecting it with tiny bubbles, planting shinier crops, building more-reflective rooftops, or thinning cirrus clouds.
While these suggestions have aroused some interest, the two most seriously investigated SRM techniques to date have been Marine Cloud Brightening (MCB) and Stratospheric Aerosol Injection (SAI) — and yes, SRM wouldn’t be considered cutting-edge science without its share of TLAs (three-letter acronyms).
MCB and SAI are arguably the most promising sun-blocking SRM schemes, more so than those aforementioned Asimov-level mirrors, not the least because such mirrors would be most effective if positioned at the first Lagrange point, an area of orbital equilibrium between the Earth and the Sun that’s a full 1.5 million kilometres away. MCB and SAI, by contrast, would work their shady magic here in our own atmosphere, and so would be much cheaper and more accessible.
That said, both MCB and SAI have problems, and some of those problems were discussed at AGU23. But before we talk about the challenges posed by those two more-realistic SRM techniques, let’s briefly define them.
Marine Cloud Brightening — MCB — is a technique to spread sea salt aerosols into select areas of the atmosphere in order to stimulate the formation of sunlight-deflecting stratocumulus clouds — which, as their strato-prefix implies, form at high altitudes. “All well and good,” you might ask, “but what are sea salt aerosols?” Okay, more definitions are in order. An aerosol, simply put, is a tiny particle dispersed in some medium, usually air or a gas. A sea salt aerosol (SSA) is, you guessed it, composed of salts from the sea, naturally generated by any number of factors, including ocean-water bubbles bursting from whitecaps or simply wind shearing them from waves. Generally, the higher the wind, the more natural SSAs are produced. However, they can also be produced industrially on giant floating barges and squirted into the atmosphere — and those are the SSAs that MCB employs.
The value of MCB’s SSAs to SRM is primarily twofold. First of all, they’re reflective. Really reflective. Really, really reflective. They essentially don’t absorb any light at all, reflecting about 97 per cent of any light that shines upon them. Secondly, even sub-micron SSAs are highly hygroscopic, which is geek-speak for their ability to attract and hold onto water molecules. Thus, SSAs both reflect sunlight and help form clouds due to their high hygroscopy, and the clouds that they help form are quite bright due to the Twomey effect, hence the SRM term Marine Cloud Brightening. In addition, SSAs keep those clouds alive longer in a process called the Albrect effect [PDF]. The most effective way to induce — produce? — such stratocumulus clouds is over the oceans, hence the term Marine Cloud Brightening.
Stratospheric Aerosol Injection — SAI — works to accomplish the same reflective task, but in a manner both similar and different.
The similarity is easy: Both MCB and SAI aim to block the sun’s energy from bouncing off the Earth’s surface, exciting those GHGs, and thus warming the troposphere. The differences are also fairly straightforward: First of all, MCB would essentially be localized; its proponents suggest it would be sufficient to inject SSAs over just five per cent of the world’s oceans in order to blunt the effects of global warming, with that injection focused most effectively, though not exclusively, in the eastern Pacific.
SAI, on the other hand, would inject its aerosols far more globally. Also, the most-studied candidate aerosols for SAI are inorganic sulfates, not MCB’s sea salt aerosols. To put it simply — very, very simply — SAI would mimic the addition of sulfates into the upper atmosphere as has historically been provided by such volcanic eruptions as Mount Pinatubo in 1991, which measurably cooled the Earth for at least two years as its sulfate contributions circled the globe after combining with water vapor to transform into sulfuric acid aerosols.
You might reasonably be thinking, “Well, if either or both of these methods can buy us some time while we wean ourselves from our addiction to GHG-spewing fossil fuels, what’s the problem? Go for it!” Well, sadly, there are some caveats that apply to both, and others that apply to each individually.
Now for the downsides
First, no SRM tech reduces the amount of GHGs that are already mucking up our ecosystems. For example, increased tropospheric CO2 contributes to ocean-acidification havoc as the Earth’s oceans suck up a goodly chunk of the CO2 that we’re pouring into the atmosphere. The resultant reduction in the ocean’s pH levels makes it more difficult for our delicious underwater friends such as clams, shrimp, oysters, and lobsters to grow their necessary shells, plus coral reefs to grow their … uh … reefs. Ecosystem smackdown.
Second, once you start SRM, you better not stop until you’ve stopped producing GHGs. Neither MCB nor SAI do squat about the production of GHGs; they only mitigate their heat-trapping nastiness. If either MCB or SAI are stopped but you’ve continued to add GHGs to the atmosphere while they were in effect, well, Katy, bar the door: All the GHGs that were added during the SRM era will cause those increased heat-trapping gasses to redouble their efforts when the Sun’s rays resume their warming of the Earth, but now they’ll be amplified by a greater concentration of GHGs in the troposphere. Insta-toastiness.
But those weren’t the already well-known effects that were troubling Chen Xing of the University of California at Santa Barbara and Antonios Mamalakis of the University of Virginia and Colorado State University during their talks at AGU23 this month. You might choose to file their insights under the all-too-familiar dolefulness of “Unintended Consequences.”
Fancy tinkering with the atmosphere? The Derecho supercomputer can advise
The alternative to stopping climate change is untested carbon capture tech
Climate change prevention plans ‘way off track’, says UN
Greenpeace calls out tech giants for carbon footprint fumble
Xing spoke of her work analyzing the effects of MCB on the El Niño Southern Oscillation, often simply referred to as El Niño or by its more proper acronym, ENSO. The effects of this natural climate-cycle, among other things, cause trade winds to push warm Pacific water east towards the west coast of the Americas — El Niño — and then reverse, and push warm water east towards Asia — La Niña.
ENSO’s effects are not merely felt locally, where they greatly affect weather and fishing abundance in both the east and west Pacific depending upon whether Niño or Niña is dominant, but more importantly in how they affect climate and weather events globally. The Pacific Ocean is huge. Its effect on the world’s climate is huge. If the ENSO cycle should grind to a halt, the effect would be equally huge.
And that’s exactly what Xing’s modeling and analyses warn might very well happen if MCB is given the green light. To explore the effects on ENSO to be expected by the two leading SRM technologies, she modeled both SAI and MCB.
To model SAI, she used the well-accepted ARISE-SAI-1.5 simulation, which dials in the amounts and locations of SAI injections necessary to keep temperatures at the oft-stated goal of 1.5°C above preindustrial levels, even if GHG continue to rise at what’s commonly known as the “middle of the road” level — called SSP2-4.5, for you lovers of initialisms.
When measuring MCB’s effect on ENSO, she used on the commonly accepted Nino 3.4 Index, which tracks sea-surface temperatures to define El Niño and La Niña events. She then modeled MCB’s future effects on that index using essentially the same Community Earth System Model 2 (CESM2) system, developed by the National Center for Atmospheric Research (NCAR) that she used for SAI.
Xing’s modeling produced two notable results. First, while MCB would indeed cause a quick and significant reduction in heat-causing solar radiation, its effect would wear off more quickly than would SAI’s. Essentially, MCB buys you more cooling effects at first, but after that its effects track essentially similarly to the “middle of the road” SSP2-4.5 increases, although beginning at a lower temperature level. Temperatures in the SAI scenario, however, remain relatively constant, even with the SSP2-4.5 increase in GHGs, with the crossover between MCB and SAI occuring about 20 years after their inceptions.
MCB’s second effect may be more troubling: It suppresses ENSO. SAI doesn’t. What’s more, MCB’s suppressive effect on ENSO doesn’t wear off as does its ability to keep temperatures down: Under MCB, temperatures rise along with GHGs, but ENSO is quickly suppressed and remains suppressed.
The reasons for this variance are complex, but they essentially involve MCB suppressing the air-sea feedbacks that produce the ENSO cycle, including reducing convection and deepening the ocean’s thermocline, the temperature boundary between the ocean’s deeper, colder zone and its warmer, more shallow zone. Under MCB, ENSO would settle into what could be described as an “eternal La Niña.”
More headaches
To make MCB matters even more problematic, modeling performed by Jack Chen of NCAR and Cindy Wang of Princeton University, also speaking at AGU23, pointed out some additional caveats. Chen’s research suggests that MCB injections as located by Xing and others could actually increase global warming in some regions, such as in Indonesia and Northern Australia. Wang’s work shows a significant slowing of wind speed in the Southern Ocean, as well as lesser but noticeable wind-speed slowing off the eastern coast of Japan and between North America and the UK.
While Xing compared MCB and SAI and their impact on just one global climate driver, ENSO — although an exceptionally important one — Antonios Mamalakis looked specifically at SAI. He, however, broadened his analysis into a wider range of global impacts.
His research, detailed in a recent paper in Geophysical Research Letters, was focused on answering a simple but subtle question. As he put it at AGU23, “How distinguishable will the SAI climate be from the pre-deployment climate?” While one might quibble with his use of the word “will” rather than “would,” it’s a good — one might even say crucial — question.
Simply put, with an equal increase in GHGs, an SAI-influenced climate might not simply be cooler than a climate without SAI. It might also be different in terms of precipitation, droughts, wind, and whatever — and before we start injecting sulfate aerosols into the stratosphere it would be good to have some idea of how distinguishable those changes might be in an SAI-influenced world from a world without SAI.
To use a technical term universally accepted among climatological phraseologists, “Well, duh …”
To accomplish his investigation, Mamalakis and his team used a proprietary tool built on “explainable artificial intelligence” (XAI), an analytical method of analysis he described as being “a new way … not necessarily better, but a new way.” (There’s a lot to be said for the inherent humility essential to the scientific method, eh?)
Going deeper
His XAI system consisted of a neural network fed with data that came from both a model that simulated a global reference climate — good ol’ SSP2-4.5 — and an SAI-influenced model simulated with ARISE-SAI-1.5. The goal was to have the network learn to discriminate whether the data came from the reference climate or the SAI climate. What was important after that learning phase was to be able to then query the XAI about how the different signals, whether they be local, global, or any combination, were distinguishable from a reference model of the climate both before SAI and without SAI. In other words, “How distinguishable will the SAI climate be from the pre-deployment climate” and from the ongoing climate without SAI, with warming increased at a rate projected by SSP2-4.5.
As Mamalakis described it, this method allowed the XAI to ferret out “the robust patterns of change that make the pre-deployment and SAI climates distinguishable.” The more distinguishable the signals were from the “reference climate” — meaning before the period during which SAI was either employed or not — the larger their variance: the more distinguishable the pre-deployment and SAI-influenced variances were from the reference climate, the better the injection of SAIs slowed climate change, and the less distinguishable their variances from the reference climate, the less effective would be squirting all those sulfates into the stratosphere.
The results were interesting. An SAI-influenced future will allow some global-warming effects to increase, but will also tamp down others. However, being a scientist and neither a marketeer nor a politician, Mamalakis was quick to point out that his XAI’s observations were not rock solid, with the SSP2-4.5 projections having an 85 per cent probability and the SAI projections weighing in globally at around 58 per cent. That word “globally,” however, is an important one: In some locations, the SAI probability was higher.
Little effect
Mamalakis and his team asked the XAI to examine over twenty different climate variables with and without SAI, and to compare each to the reference model. Some variables were similar enough to be called essentially indistinguishable from the reference period: ocean heat content and ocean acidity, for example, were highly correlated between the SSP2-4.5 projection and the SAI projection, meaning that the injection of SAI had little effect.
What was perhaps more interesting were the XAI data that showed a divergence between “middle of the road” global warming as predicted by SSP2-4.5 and a future influenced by SAI. In these cases, the future effects of those two scenarios are quite distinguishable: drought frequency and severity, precipitation over both land and the oceans, and the extent of Arctic ice. Other climate science shows that those changes are amplified by climate change; Mamalakis’ work shows they would be mitigated by SAI.
Shame about those wildfires. We’ll just let the fossil fuel giants off the hook, then?
READ MORE
Also of interest was the regionality of some of the differences between the SAI and non-SAI futures. In general, global temperatures would be kept significantly close to those of the reference model if SAI is employed, but that cooling would not be globally consistent. For example, annual average temperatures would be much cooler over the north Atlantic, and maximum temperatures would drop in the middle latitudes of the southern hemisphere — think Argentina and eastern Australia. In addition, although annual precipitation and the length of droughts won’t change much globally in an SAI-infused future from what we currently experience, regional drying-out would increase in the Amazon and tropical Africa.
What the work of Xing, Mamalakis, and their colleagues shows is that although geoengineering — specifically Marine Cloud Brightening and Stratospheric Aerosol Injection, in their case — holds promise in slowing global warming during the transition to clean energy, there’s still much to be learned about the regional effects of any form of such tinkering. Tweaking Earth’s climate systems in response to global warming–induced climate change is a classic example of what’s known as a “wicked problem” — meaning one that is hard to articulate and can’t be solved in any simple way or in a way that has a definitive ending. Global-warming mitigation and adaptation is a fiendishly complex process, not an open-and-shut case of: “Just do this and all will be well.”
Luckily, the research of Xing, Mamalakis et al is only a fraction of what is being done globally by the many climate scientists who are investigating geoengineering. The scope of that effort is welcome, as there’s still much to be learned before mankind embarks on a course as radical as attempting to reverse climate change through solar radiation management — if, in fact, it does so at all.
That said, each and every one of the AGU23 sessions that focused on global warming, climate change, geoengineering, and other such matters did bring to mind an irrefutable fact, one that was summed up in a margarine commercial some fifty years ago: “It’s not nice to fool Mother Nature.” ®
Bootnote
Your humble Register correspondent thought he was well defended against the onslaught of any and all climate-science acronyms until he was forced to deal with ARISE-SAI, a tortured rendition of “Assessing Responses and Impacts of Solar climate intervention on the Earth system with Stratospheric Aerosol Injection”. He hereby surrenders unconditionally.